TIFRH researchers uncover a mechanism enabling glasses to self-regulate their brittleness
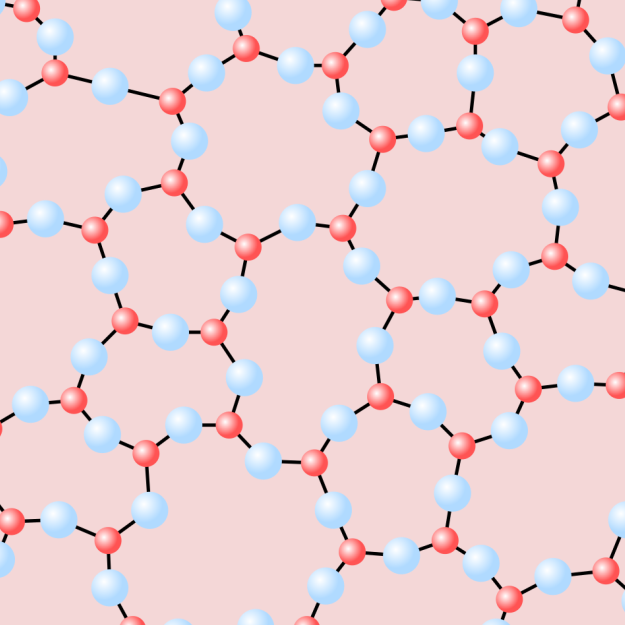
Materials with self-adaptive mechanical responses have long been sought after in material science. Using computer simulations, researchers at the Tata Institute of Fundamental Research (TIFR), Hyderabad now show how such adaptive behavior can emerge in active glasses, which are widely used as models for biological tissues. The findings provide new insights—ranging from how cells might regulate their glassiness to aiding in the design of new metamaterials.
Glasses (or amorphous solids) are materials whose components lack any particular ordering. Contrast this with a crystal, where atoms are arranged in neat, repeating patterns on a well-defined lattice. While crystals are ordered and nearly perfect, amorphous materials are defined by their disorder.
When such disordered materials are composed of components that can utilize internal energy reserves and move autonomously, they form what are known as active glasses. Such systems are abundant in environments where particles are tightly packed and also possess chemical reserves—for instance, epithelial cells or bacterial colonies.
Not all glasses are created equal, however. Depending on their preparation, the same material can form glasses with widely varying mechanical properties. This history dependence is a hallmark of such non-equilibrium systems—they have a memory of how they were prepared!
To prepare a glass, start with a liquid (almost any liquid will do!) and cool it rapidly past its melting point. With fast enough cooling, crystallization can be avoided, resulting in a supercooled liquid. As the temperature continues to drop, there is an empirically (or experimentally) defined temperature below which the dynamics slow down so dramatically that tracking the system in any detail becomes infeasible. This is the famous glass transition temperature. Below this point, the system is effectively in an arrested state. And voilà! you have a glass.
What is interesting is that although the dynamics have changed drastically, structurally, the system hasn’t changed much. A clear understanding of how this crossover to an arrested state occurs is still lacking. Incidentally, the nature of this transition was once referred to by Nobel Laureate P. W. Anderson as “the deepest and most interesting unsolved problem in solid-state theory” [Science, 1995]—and it still remains elusive. Depending on how quickly or slowly you cool across this glass transition temperature, you get glasses with different mechanical properties. Generally, slower cooling results in well-annealed glasses, which are brittle (systems that break abruptly with a sharp snap under external loading), whereas faster cooling result in poorly annealed glasses, which are more ductile (systems that elongate and form necks before eventually breaking).
Another outlooks for visualizing this is that of an energy landscape, with barriers (hills) separating local minima (valleys). Any glass configuration can be thought of as being stuck in one of these many local minima, with barriers preventing it from transitioning and exploring lower energy states. Better cooling while creating the glass leads the system to a deeper minimum in this landscape.
Sharma and Karmakar discovered that taking a poorly annealed glass and imparting additional motility to some fraction of its components induced further annealing in the system, taking it to lower and lower regions of the landscape. This is reflected in the system’s potential energy, which gradually decreases. They showed that the local rearrangements caused by activity in a glass can anneal the system enough to make an initially ductile material brittle. In essence, the researchers have found that active dynamics provide a means to traverse the energy landscape more effectively.
What was even more interesting was that the phenomenon of annealing through local perturbations seemed to parallel how glasses behave under repeated cyclic shear deformation. To briefly explain shear deformation: Imagine taking a solid box and fixing its base so that it cannot move. Now, hold the top and push and pull it along a fixed direction parallel to the top face. This is an example of oscillatory shear deformation.
Extensive rheological studies have been conducted on various materials using both simple (one-directional) and oscillatory shear.
This study found that imparting activity to a fraction of the glass particles resulted in many of the same phenomena observed when a glass is subjected to oscillatory shear. For instance, one characteristic of glasses under oscillatory shear is that if the oscillations are performed below the yielding amplitude (i.e., the extent to which the system is deformed), the amplitude somehow gets imprinted onto the system. This imprint can later be “read” out using sophisticated techniques. Remarkably, similar memory effects were also discovered in the case of active glasses, using slightly different but analogous reading protocols.
Other similarities included how the system could transition from a stuck state to a fluidized state at larger driving values. This fluidization in active glasses has been extensively studied in the literature and is crucial for understanding the biophysics of wound healing and morphogenesis, both of which involve mass cell migration.
The fact that a system as complex as an active glass can essentially be mapped onto an ordinary glass under oscillatory shear allows for a better understanding and provides new insights into the nature of active glasses, in terms of a much simpler and extensively studied system.
While this study establishes internal activity as a means to anneal glasses, it still can’t compete with in-silico annealing techniques like Swap Monte Carlo, where non-physical moves—such as swapping two particles over arbitrary distances—are allowed. Further exploration is needed to determine whether such local methods can be made more competitive with dedicated annealing techniques like Swap Monte Carlo or whether they could be used in tandem to equilibrate glasses even further. These questions present exciting avenues for future research.
Content: Rishabh Sharma, EurekAlert release: 17-05-2025
Publication reference: Sharma, Rishabh, and Smarajit Karmakar. “Activity-induced annealing leads to a ductile-to-brittle transition in amorphous solids.” Nature Physics (2025): 1-9.
Media Mentions: Phys.org, Bioengineer.org
Nature News and Views Article Thomas Voightmann which features this study: The amorphous materials behind biophysics
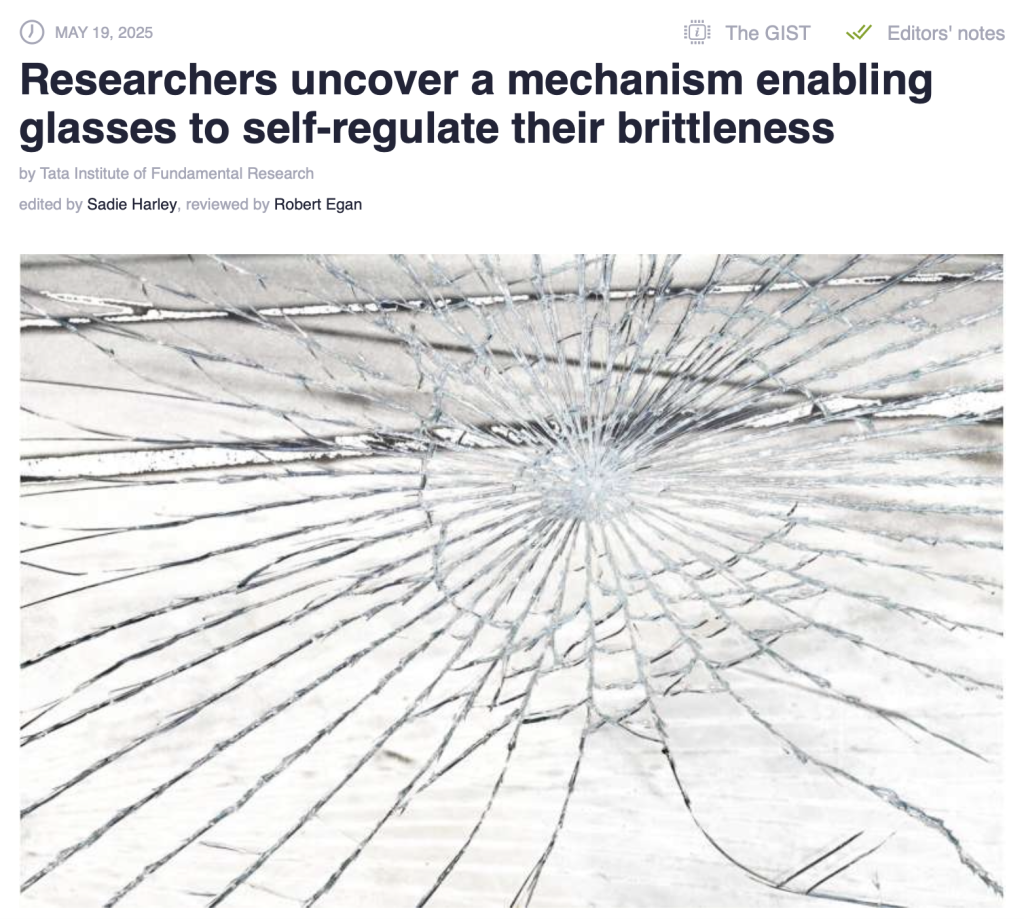
Image: Coverage of the study on Phys.org